The Role of the Nephron in Regulation of Urine Volume and Osmolality
The kidneys are the fundamental organs of the excretory system. Their main functions are the excretion of products of metabolism, hydro electrolytic regulation, osmolarity and body acid-base balance, blood pressure, hormone secretion, and gluconeogenesis. Most of these tasks are performed through the filtration of blood plasma which provides the filtrate to be processed to form urine that will be excreted through the urethra.
In the kidneys, urine is formed in the nephrons, which constitute the smallest filtering units of these organs. Nephrons are the functional structures where blood filtration and urine formation occur. The primary structure of a nephron entails having a renal corpuscle and a tubule where the corpuscle is the Bowman’s capsule while the tubules include proximal convoluted, loop of Henle, distal convoluted, and collecting ducts. Both the right and the left kidney consist of 600000 to 800000 nephrons that allow them to filter urine from blood (Lawrence et al., 2018). Nevertheless, the ultrafiltration process occurs at the glomerulus, situated at the Bowmans capsule (Figure 1). The glomerulus is highly vascularized to increase the surface area for diffusion of blood from the glomeruli capillaries into the Bowman’s capsule. When viewed under an electric microscope, podocytes, which entails cells that throw their membranous projections to toe glomeruli, can be seen since they are the ones that allow the passage of substances through the tiny pores they leave open. The process of urine formation starts immediately after the filtrate enters the Bowman’s capsule.
Mechanisms of Urine Osmolality and Volume Regulation
Urine volume and osmolality are regulated depending on the body’s water needs. During exercise or on hot sunny days, the body loses water via sweating and increased breathing rate, meaning a sense of dehydration occurs. Water reabsorption in Henle’s descending loop rises to compensate for the lost water. The nephrons can integrate the endocrine system through vasopressin’s involvement, which stimulates the distal convoluted tubules and the collecting ducts to reabsorb more water. The osmolality of the urine rises while the level of water decreases, leading to the production of less amount of concentrated urine.
The nephron contains a group of anastomosing capillaries called the glomerular capillaries, forming the glomerulus. These capillaries are fenestrated, meaning they have holes in their walls. Due to these fenestrations, the plasma is filtered through the endothelium of these capillaries, passing through two more layers: the basement membrane and the podocytes, epithelial cells resembling tentacles that envelop the glomerulus (Theodorou et al., 2021). Then there is a space separating the glomerulus’s outer leaflet from Bowman’s capsule’s inner wall, called the capsular space. The capsule is an envelope that receives the ultrafiltrate and directs it to the proximal convoluted tubule, where the processing of the tubular fluid begins.
The proximal tubule gives rise to the loop of Henle that plunges into the renal medulla and has three segments: thin descending, thin ascending, and thick ascending; only animals that have this structure can concentrate urine (Theodorou et al., 2021). As it ascends, the loop of Henle passes between the afferent and efferent arterioles of the glomerulus. This point is where the juxtaglomerular apparatus and the distal convoluted tubule, which precedes the collecting tubule, begin. It flows into the collecting duct, a larger structure that collects the filtrate from several collecting tubules and penetrates the renal medulla, emptying its contents into the orifices above the renal papilla.
The kidneys control salt concentrations in tissues and cells and ensure excretion and osmoregulation. They ensure body fluid balance by removing products of cellular metabolism and chemicals found in extremely high concentrations in the body. The kidney ensures that these products are removed by forming urine in the nephrons. The glomeruli are under great pressure, causing a portion of the blood plasma to exit and travel into the glomerular capsule. The ultrafiltrate, comparable to plasma, is created during the filtration process. The filtered fluid subsequently passes into the nephric tubule, where chemicals are reabsorption and released, completing the urine production process. Therefore, the nephron controls the amount of fluid, water, and salts in the blood, enhancing the body’s osmolarity and urine volume. The nephron excretes creatinine, urea, and excess water to make up urine in excretion.
The regulation of renal function relates to regulating the amount of fluid in the center and extracellular compartments. To retain more water inside the body, the urine becomes more concentrated due to greater water reabsorption (Theodorou et al., 2021). In this case, hyperosmolarity exists, where the amount of water is low in the plasma, causing the water to go to the LEC. However, the urine becomes less concentrated when excess water is in the body due to less water reabsorption. In this case, hyperosmolarity exists, where the plasma is filled with water, causing the water to go to the LIC to compensate for this imbalance.
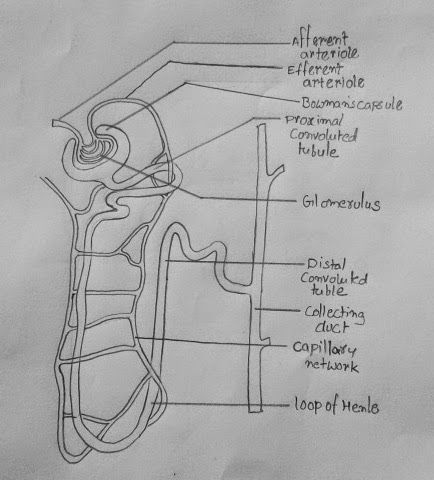
Effect of Autonomic Nervous System on the Cardiac Output
Sympathetic Nervous System
Cardiac output is the volume of blood emitted by the left ventricle into the aorta artery per minute. The cardiovascular system is a closed system. There should be no losses, so the cardiac output must equal the venous return, the volume of blood that reaches the right atrium every minute in an efficient heart. On average, 5,250 ml/min are ejected cardiac output, and the same amount must return to the right atrium, venous return (Borovac et al., 2020). The heart is innervated by the autonomic nervous system and receives fibers from the sympathetic nerves. Typically, cardiac output is calculated by multiplying the heart rate with the systolic blood volume. Therefore, an increase in one or both factors increases cardiac output. Upon stimulation of the cardiac muscles by the sympathetic nervous system, the force, and speed of contraction increase, leading to an increase in the heart rate, which ultimately means the amount of blood ejected by the heart rises based on volume.
Nerves from the spinal cord form nerve ganglia parallel to the cord far from the organs, innervating the Sympathetic Autonomic Nervous System. Nerve nuclei – clusters of nerve cells – of the sympathetic system are found in the thoracic and lumbar regions of the spinal cord (Borovac et al., 2020). The spinal cord nerves anterior roots are located in axons from where neurons escape. Sympathetic division nerves typically emit noradrenaline, which enhances alert-related reactions such as increased heart rate, breathing rate, and pace.
The noradrenaline substance released by the sympathetic nervous system is distributed to the heart’s cardiac muscle, increasing the rate of heartbeats. When the heartbeats increase, the ventricular activity increases (Badke et al., 2018). Moreover, the lumen of the blood vessels is reduced; hence blood is pumped under high pressure leading to high cardiac output. The sympathetic nervous system activation results in a lower volume of the ejected blood, systolic output, due to increased pressure in the aorta artery, making it difficult to eject blood from the left ventricle. A higher sympathetic tone causes tachycardia or heart rate acceleration. In addition, the increase in sympathetic tone causes greater secretion of catecholamines, epinephrine, and norepinephrine, by the adrenal medulla, which contributes to an increase in heart rate (Badke et al., 2018). An increase in stroke volume promotes an adaptive response of the heart that includes an increase in its contractility. Hence an increase in sympathetic tone promotes greater secretion of the adrenal gland’s medullary zone hormone, adrenaline. The beta 2 effect is a vasodilator, and the alpha effect is a vasoconstrictor. Nor-adrenaline, also from the adrenal gland, has only a vasoconstrictor effect.
Before assimilation with the sympathetic trunk, sympathetic fibers begin in the intermediolateral cell column of the thoracic cord, between T1 and T5. Preganglionic fibers make synapses in the sympathetic ganglia of the cervical and thoracic regions, and postganglionic fibers reach the cardiac plexus (Vaillancourt et al., 2018). Sympathetic innervation works in opposition to the parasympathetic system, raising heart rate, cardiac contractility strength, and coronary artery dilatation. Because heart rate is a function of cardiac output, increasing the amount of blood ejected by the heart during systole is the end outcome.
Parasympathetic Nervous System
The activation of the parasympathetic nervous system happens when the sympathetic nervous system is deactivated. The activation of the parasympathetic nervous system deactivates the atrioventricular and sinoatrial nodes, therefore, lowering the heart rate. Parasympathetic fibers originate from the vagus nerve and cranial nerve X (Vaillancourt et al., 2018). They are classified as preganglionic fibers synapse with postganglionic neurons, either within a part of the cardiac plexus or within the heart wall itself. Unequivocally, the main role of the parasympathetic nervous system is to lower the heart contraction by producing inhibitory effects to the sympathetic stimulation via the release of acetylcholine, thus lowering the heart rate and the cardiac output.
The activation of the parasympathetic nervous system results in temporary but practical reflex effectiveness. It results in the generation of the baroreflex, the basic short-term regulatory mechanism. Located in the carotid sinuses and the aortic arch are receptors that permanently inform the CNS about the blood pressure through afferent neural pathways (Vaillancourt et al., 2018). This information is received by the nucleus of the solitary tract in the medulla, and the effector response occurs through a cardiomoderator, parasympathetic, region. The parasympathetic system has no generalized vasodilating action and acts on some organs.
Stimulation of baroreceptors occurs with variations in blood pressure, between 60 and 200 mmHg in humans. To exemplify the reflex action of the baroreceptors, transition to the orthostatic position is established when there is intense venous blood sequestration in the lower limbs and, therefore, the reduction in systolic volume and blood pressure (Badke et al., 2018). With the detection of the alteration by the receivers in question, there is reflexively a decrease in the discharge frequency and, consequently, the stimulation of the centers.
Moreover, the activation of the parasympathetic nervous system causes the heart to generate its electrical impulses that follow its path, specially designed to help distribute an action potential through the heart muscle (Figure 2). The nodes and networks of the heart’s “specialized” cells make up the cardiac conduction system (Badke et al., 2018). This system constitutes atrioventricular and sinoatrial nodes with the subendocardial plexus that control the conduction of ventricular cells. The pacemaker rhythm of the heart is generated at the level of this system, influenced by nerves and transmitted from the atria to the ventricles and, from there, to all the musculature (Badke et al., 2018). The activation of the parasympathetic nervous system deactivates the sinoatrial and atrioventricular nodes resulting in a decrease in heart rate, widening of the blood vessels, vasodilation, and decrease in the heartbeats. Therefore, all these events reduce the overall volume output of blood in the aorta, hence low cardiac output.
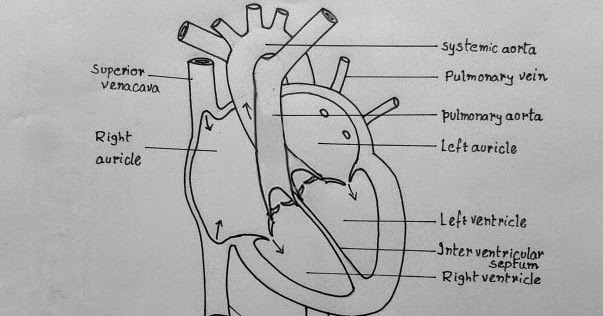
References
Badke, C. M., Marsillio, L. E., Weese-Mayer, D. E., & Sanchez-Pinto, L. N. (2018). Autonomic nervous system dysfunction in pediatric sepsis. Frontiers in Pediatrics, 6, 280.
Borovac, J. A., D’Amario, D., Bozic, J., & Glavas, D. (2020). Sympathetic nervous system activation and heart failure: Current state of evidence and the pathophysiology in the light of novel biomarkers. World Journal of Cardiology, 12(8), 373.
Lawrence, E. A., Doherty, D., & Dhanda, R. (2018). The function of the nephron and the formation of urine. Anaesthesia & Intensive Care Medicine, 19(5), 249-253.
Theodorou, C., Leatherby, R., & Dhanda, R. (2021). The function of the nephron and the formation of urine. Anaesthesia & Intensive Care Medicine, 22(7), 434-438.
Vaillancourt, M., Chia, P., Sarji, S., Nguyen, J., Hoftman, N., & Umar, S. (2018). Autonomic nervous system involvement in pulmonary arterial hypertension. Respiratory Research, 18(1), 1-15.